Wednesday, 13 September 2017
Scientists create alternate evolutionary histories in a test tube
in: Biology Evolution GeneticsScientists at the University of Chicago studied a massive set of genetic variants of an ancient protein, discovering a myriad of other ways that evolution could have turned out, and revealing a central role for chance in evolutionary history.
published this week in Nature by UChicago graduate student Tyler Starr and Prof. Joseph Thornton, is the first to subject reconstructed ancestral proteins to deep mutational scanning—a state-of-the-art technique for characterizing massive libraries of protein variants. The authors’ strategy allowed them to compare the path that evolution actually took in the deep past to the millions of alternative routes that could have been taken, but were not.
Starting with a resurrected version of an ancient protein that evolved a new function some 500 million years ago—a function critical to human biology today—the researchers synthesized a massive library of genetic variants and used deep mutational scanning to analyze their functions. They found more than 800 different ways that the protein could have evolved to carry out the new function as well, or better than, the one that evolved historically.
The researchers showed that chance mutations early in the protein’s history played a key role in determining which ones could occur later. As a result, the specific outcome of evolution depended critically on the way a serial chain of chance events unfolded.
“By comparing what happened in history to all the other paths that could have produced the same result, we saw how idiosyncratic evolution is,” said Tyler Starr, a graduate student in biochemistry and molecular biology, who performed the paper’s experiments. “People often assume that everything in biology is perfectly adapted for its function. We found that what evolved was just one possibility out of many that were just as good, or even better, functionally than what we happened to end up with today.”
Molecular time travel
Over the last 15 years, Thornton, senior author on the new study and a professor in ecology and evolution and human genetics, led research that pioneered “molecular time travel” using ancestral protein reconstruction. In 2013, his team resurrected and analyzed the functions of the ancestors of a family of proteins called steroid hormone receptors, which mediate the effects of hormones like testosterone and estrogen on sexual reproduction, development, physiology and cancer. The body’s various receptors recognize different hormones and, in turn, activate the expression of different target genes, which they accomplish by binding specifically to DNA sequences called response elements near those targets.
Thornton’s group inferred the genetic sequences of ancient receptor proteins by statistically working their way back down the tree of life from a database of hundreds of present-day receptor sequences. They synthesized genes corresponding to these ancient proteins, expressed them in the lab and measured their functions.
They found that the ancestor of the family behaved like an estrogen receptor—recognizing only estrogens and binding to estrogen response elements—but during one specific interval of history, they evolved into a descendant group capable of recognizing other steroid hormones and binding to a new class of response elements. The researchers found that three key mutations before the emergence of vertebrate animals caused the ancestral receptor to evolve its ability to bind to the new target sequences.
That work set the stage for the current study. Knowing precisely how evolution played out in the past, Thornton’s group asked: Was this the only evolutionary path to evolving the new function? Was it the most effective one, or the easiest to achieve? Or was it simply one of many possibilities?
Alternate histories
Starr began working on the project during his first year as a graduate student, developing the technique to assess massive numbers of variants of the ancestral receptor for their ability to bind the new response element. First, he engineered strains of yeast in which the ancestral or new response elements drive expression of a fluorescent reporter gene. He then synthesized a library of ancestral proteins containing all possible combinations of amino acids at the four key sites in the receptor that recognize DNA—160,000 in all, comprising all possible evolutionary paths that this critical part of the protein could have followed—and introduced this library into the engineered yeast. He sorted hundreds of millions of yeast cells by their fluorescence using a laser-driven device, and then used high-throughput sequencing to associate each receptor variant with its ability to carry out the ancestral function and the new function.
Most of the variants failed to function at all, and some maintained the ancestral function. But Starr found 828 new versions of the protein that could carry out the new function as well, or better than, the one that evolved during history. Remarkably, evolution could have accessed many of these even more easily than the historical “solution,” but it happened not to, apparently wandering around the space of possible mutations until it arrived at the version of the protein in our bodies today.
“We all share the same gene sequence for this protein, so it might seem like evolutionary destiny, as if we’ve arrived at the best possible version. But there are hundreds of other directions that evolution could just as well have taken,” Thornton said. “There’s nothing special about the history that happened, except that a few chance steps brought us to this singular chance outcome.”
Thornton said that deep mutational scanning will be a powerful tool for evolutionary biologists, geneticists and biochemists, and he looks forward to using the approach on successive ancestors at different points in history to see how the set of possible outcomes changed through time.
“We have a molecular time machine to go back to the past, and once we’re there, we can simultaneously follow every alternate history that could possibly have played out,” Thornton said. “It’s a molecular version of every evolutionary biologist’s dream.”
Author: Matt Wood | Source: University of Chicago Medical Center [September 13, 2017]
About Admin of the Blog:
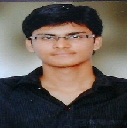
Imran Uddin is the founder of AllTechBuzz .He is a Tech Geek, SEO Expert, Web Designer and a Pro Blogger. Contact Him Here
0 comments:
Post a Comment